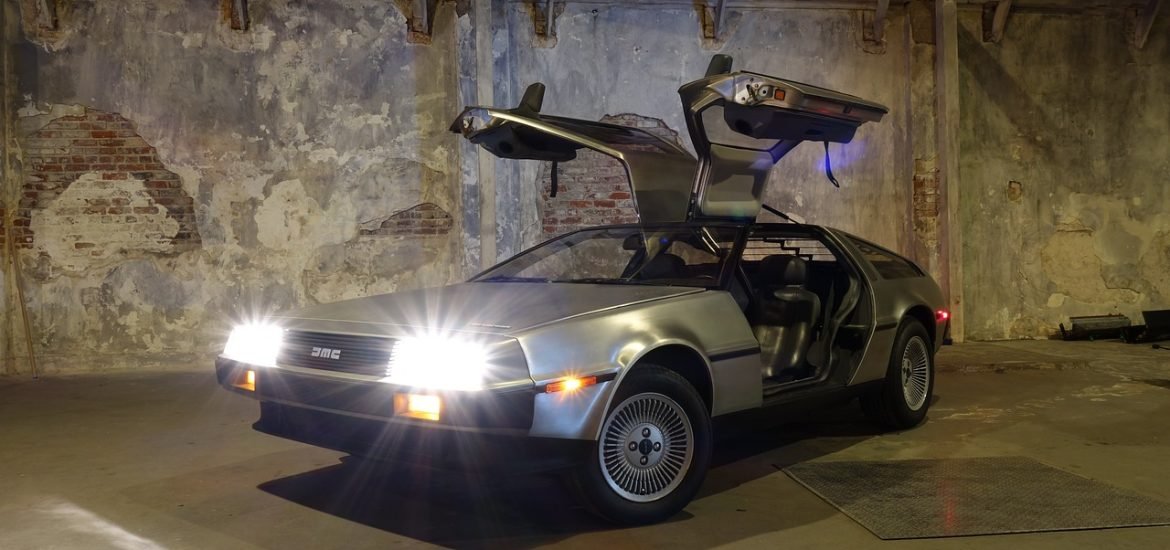
Part Two: Can technological progress break the deadlock by 2040?
“Incremental” improvements or breakthrough innovations?
The question that arises is: “Can battery technology be advanced to address its current shortcomings, and if so, by the 2040 deadline set by the legislator?”
The answer is “probably not”. This does not mean that it is impossible, but it seems unlikely for 2040. This will only be possible if a “technological breakthrough” occurs. But by their very nature, technological breakthroughs are unpredictable.
The invention of the internal combustion engine at the end of the 19th century clearly constituted a technological breakthrough. But it would have been difficult to predict before 1870, when politicians were still wondering how to manage the exponentially increasing volume of horse manure in their cities. And its adoption did not happen overnight: its progress from invention (patented by Otto in 1876) and its use, first in agricultural machinery, then in cars, was to take over 15 years. But the long-term consequences of this invention were incalculable, and led to a form of societal development that no politician could have predicted.
All of which leads us to conclude that we are able to imagine, albeit not to a very high level of precision, what the world will look like as a result of “incrementally” improving existing technologies, but that it is impossible to predict a technological breakthrough, and to base a policy on the expectation of such a breakthrough.
How quickly could we see improvements in Li/ion batteries?
Contrary to popular belief, batteries are not a new industry. The concept is 200 years old and the first lithium/ion battery prototypes were tested in the 1970s. Fewer than a dozen of the hundreds of battery types that have been tested have reached the industrial stage, and even then, they have not all been commercial successes.
And if one thing is certain after all this time, it is that there is no “Moore’s law” (source) for batteries, so we are not likely to be able to anticipate performance increase at the speed microprocessors have developed just by incremental evolution of the technology.
Before the introduction of Li/Ion batteries, the improvement in energy density of older generation batteries was capped at 3% per year.
Since Li-Ion reached the industrial stage in 1990, the average capacity gain has been around 5% per year, a 3.5-fold increase in 25 years, with a slowdown in improvement since 2010 (see graph below, source).
5% per year is equivalent to a doubling of capacity every 14 years. That is good, but even assuming we can continue at the same rate, it would result in batteries of around 1.6 MJ/kg in 2040. Which is still 28 times less dense than a fossil fuel. Such progress would be admirable, but not enough to eradicate all EV shortcomings.
Evolution of Li/ion technology costs
It is difficult to predict how battery costs will develop, but according to various analysts aggregated by Bloomberg (source), a target cost of around $62 (€55) per kwh by 2030 is a realistic projection, roughly 4 times less than today. A battery of 80 Kwh (twice the current zoe), providing “sufficient” autonomy (leaving aside any question of recharge time) could therefore be around 4400 Euros excluding VAT, roughly half of the current price. The situation would therefore be better than today, but far from being competitive with internal combustion engines, if the latter were to be allowed to continue their progress, as we will see below.
New developments: far from maturity
Technologies that “incrementally” improve existing batteries are well underway at the R&D stage, but no miracles are to be expected by 2040. No existing technology or technology in the initial laboratory research phase seems realistically capable of replacing the benefits of fossil fuels within 20 years. Fred Schachter of the Lawrence Berkeley laboratory explains (link) that the optimism that prevailed in the sector at the beginning of the century disappeared at the beginning of this decade, when many research fields proved to be less promising than initially expected.
The laws of physics and chemistry limit the theoretical energy density of Li/ion batteries to around 2 MJ/kg, or 4 times their current energy density. But we are still far from knowing how to bring the actual density of these batteries further towards their theoretical maximum.
Replacing lithium (or other currently used materials such as cobalt) with other materials is being considered, but lithium is already one of the lightest elements in the periodic table: the tests carried out often result in heavier batteries. Other researchers have developed theoretical concepts using other principles, such as zinc, silicon, or so-called “solid” electrolytes based on polymer gels, or super-capacitors. Here again, the theoretical density could be around 5 MJ/kg, but in practice, the first versions, if they are ever produced, will be around 1MJ/kg, roughly double the current Li/ion.
And to date no one knows how to move from the theoretical model to the physical model, let alone the industrial model, for any of these “super technologies”.
In search of the ideal battery
But what would actually be the specifications of an ideal battery? The energy density, which is essential for the battery to be compact and lightweight, is not the only important criterion.
- It should also be able to recharge quickly without losing performance, be tolerant to overload, and be able to deliver its energy slowly or quickly.
- It must maintain almost constant performance after each charging cycle, operate both in extreme cold and extreme heat, be easy to cool, not ignite, not explode, not run down when the car is not operating.
- It should tolerate the level of vibration or impact encountered in a car, should not cause toxic pollution in the event of an accident.
- Its manufacturing process should be “ecologically sustainable”, use little energy throughout its manufacturing and recycling cycle, and require no maintenance…
- And all this should be possible at a reasonable production cost, without using ultra rare or very difficult to process materials.
Well, to date, no battery technology, either actual or still in the laboratory, meets all these qualities.
In fact, a battery may have some of the above qualities but not all of them. For example (see diagram below), if you create a battery that can store “a lot” of energy, chances are it will not be able to release it quickly.
And the closer a battery concept comes to an operationally usable compromise, the more expensive it is, or gets a disqualifying score on a single key point (for example: industrial feasibility, the ecology of manufacturing processes, or fire stability). We are therefore still far from imagining what series of commercially viable batteries will succeed the current Lithium/ion generation.
The media dream of the “miracle battery”
Let us keep in mind that between their first laboratory test (around 1975, in England) and their initial market launch (1990 by Sony), Lithium Ion batteries required 15 years of research and development at different stages. To hope that a “miracle” technology could be in production by 2040, it would have to appear in the laboratory very soon. Knowing that it may take several years between these advances being developed and their appearance in the media, it is impossible to say that no such discovery is ongoing. But nothing for the moment suggests otherwise!
The Internet regularly brings us breaking news that “researchers have found a miracle material to create revolutionary batteries.” But as Professor Jud Virden, director of a leading battery research laboratory, said in a testimony before the US Congress in May 2015 (link),
“You see all kinds of press releases about a new anode material that’s five times better than anything out there […] and it probably is, but […]when you put that in with an electrolyte and a cathode, and put it together and then try to scale it,
all kinds of things don’t work. Materials start to fall apart, the chemistry isn’t well known, there’s side reaction, and usually what that leads to is loss of performance, loss of
safety. And we as fundamental scientists don’t understand those basic mechanisms.
…. And we do really undervalue the challenge of scale-up… in an experiment in a lab like this big, it works perfectly. Then when you want to make thousands of them -it doesn’t.”
In short, beware of the effects of media hype about laboratory discoveries! From the laboratory to industrial application, the road is full of pitfalls.
Spending more money on the “batteries” sector will not create a miracle.
Currently, the battery cell giants, Samsung, Panasonic and LG, are investing mainly in incremental improvements to their existing Li/ion batteries rather than in new research avenues. Even Tesla, who assembles cells produced by the above 3, but hasn’t developed a cell itself, is pursuing this conservative approach. Why? Because no “winning sector” promising to move beyond the Li/ion era has emerged, and betting on 15 years of R&D without a guarantee of success seems less promising to them than improving what already exists… Especially since the existing one is heavily subsidized!
A 2016 study of more than 4 billion invested in North American start-ups in the sector (source) showed that on average, they obtained 40 million over 8 years, while Tesla is ready to put 5 billion on the table in its “conventional” battery gigafactory. Clearly, these sums are not sufficient to move from the “promising anode material” stage to the “prototype battery candidate for industrial application” stage. And apparently none of these alternative avenues has to date managed to convince a major industry player to back them.
Wrong-headed good ideas: charging by replacing the battery pack
For the EV user, any shortcomings in autonomy would not be a big problem if recharging could be fast, hence the idea, considered briefly by some manufacturers, of having pre-charged battery packs at petrol stations, which could be changed on demand and recharged “in their own good time” by the supplier.
Unfortunately, this idea, attractive on paper, quickly comes up against complex practical and logistical considerations, and therefore prohibitive cost issues.
First of all, the battery packs would have to be relatively standardised, as well as their location on the vehicle (or in a trailer), so that the service station did not have too many lines to stock. After all, they would need to benefit from large stock volumes. On the other hand, the economic efficiency of these petrol stations presupposes that they can turn over their battery stock very quickly. This means that it is necessary to be able to recharge at very high speed at any time of the day, including at times when electricity will be most expensive.
But fast and frequent charging of batteries also means faster degradation of their performance. Also, the motorist would not know if he was receiving a new pack with its full load capacity, or a used pack giving him less real autonomy. And finally, on busy holiday period days, the system would certainly be either overloaded or undersupplied. In short, there are still many unknowns about the real feasibility of this model, both technical and economic.
In 2012, D. Hillebrand, director of the energy department of a large US national laboratory, stated (source) that as things stood, batteries were 20 times too expensive for a battery exchange business to be viable. Since then, the price of batteries has been cut to about a third, and a further cut to a quarter is expected by 2030. In ten years’ time, they would therefore still be nearly 2x more expensive than necessary to enable profitable business models without subsidies. Mr. Hillebrand’s statement was reinforced in 2013 by the massive failure of this model tested by Renault on the Israeli market.
Hydrogen: this is not the miracle you are looking for
What about hydrogen? In theory, its energy density by weight is 3 times higher than that of fuel oil (source). So, is this the ideal fuel source? No! If the density relative to weight is good, the density by volume is disastrous, because hydrogen is a gas!
Even compressed at 700 bars, the “volume” energy density of hydrogen is 6 times lower than that of fuel oil. And hydrogen production is itself quite energy-intensive. And the list of characteristics of hydrogen powertrains is still far from meeting all the criteria of the specifications described above for “ideal” batteries, starting with their currently prohibitive cost. The potential of hydrogen should not be minimized, but to see it as the dominant pathway in 2040 is premature.
This post is also available in: FR (FR)DE (DE)
Keep up the good work. the California govt is banning gasoline cars. The problem is climate change communists.