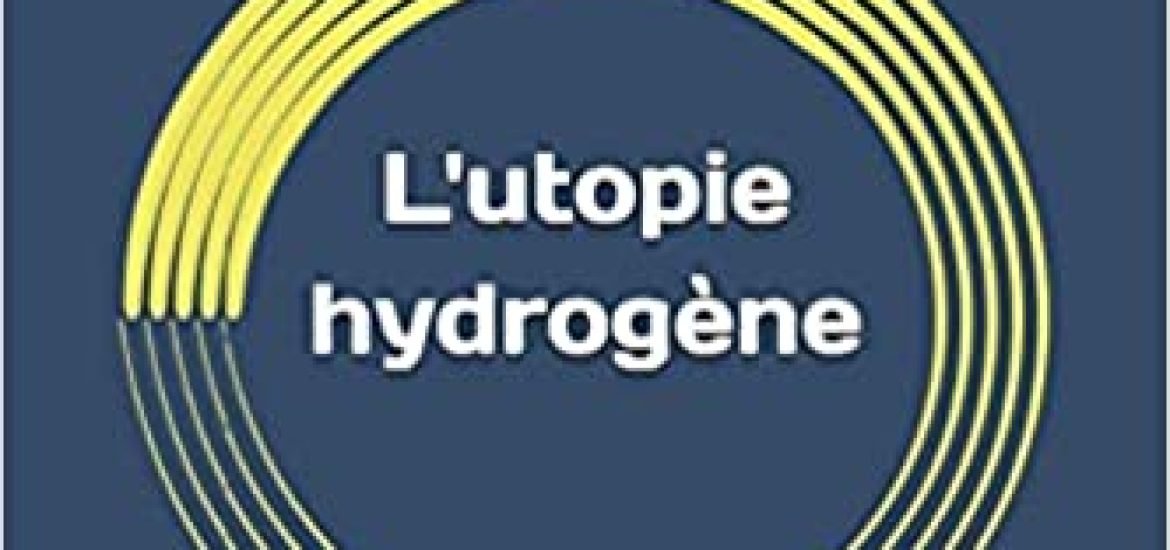
Samuel Furfari[a] and Henri Masson[b] review the possibilities allowed by hydrogen and the EU’s policies
The enthusiasm for hydrogen
Hydrogen suddenly seems to have become an exceptional solution for the energy transition, also called decarbonisation of the economy. Not a day goes by without an industry, an authority or media announcing more and more extraordinary uses. We are assured that motor cars, trucks, planes, boats will move with hydrogen. We will even heat our homes with this molecule, and they say that the steel industry will run with hydrogen, itself produced by electricity generated by wind turbines. Whilst hydrogen is burning, it is understandable that some people are thinking about it, especially since the EU has announced that the hydrogen strategy is becoming a central point of its decarbonisation policy. The European Commission will also devote 30% of the loan that it takes out for helping with post-crisis recovery linked to the pandemic, to the fight against climate change . Of this 225 billion euros, part of it, left to the choice of the Member States, will be used to try and promote the emergence of hydrogen as an energy vector.
Of course, as it is supposed to be a solution to the energy transition, the production of hydrogen energy must be made from renewable energies. However, since biomass and hydropower are under increasing attack by environmental movements, it is clear that the EU’s target of almost 100% of decarbonised energy for 2050 will be based on wind and solar energy, despite their variable, unpredictable, and intermittent re-inhibiting nature.
This makes today, but also tomorrow the green hydrogen solution impossible in an open and competitive world. This is also a no-brainer for Germany. Recently in an article entitled ‘CO2 must become more expensive,’ the Frankfurter Allgemeine Zeitung reported that the German Minister of Research, Anja Karliczek, and the CEO of Siemens Energy, Christian Bruch, acknowledge that the deployment of green hydrogen technology will depend on regulation and legislation, and ‘not so much on scientists’.
This means admitting that, from a scientific and technological point of view, the hydrogen strategy makes no sense. Green hydrogen will only become a fuel if required by law. Of course, this is always possible, but then you can be sure that we will be in an EU fortress, cut off from the rest of the world. In a free, open and competitive world, imposing hydrogen as a fuel is simply stupid. We will show in this article why these two German personalities, and we too, believe that only legislative constraint can impose the use of hydrogen as an energy vector.
The inevitable intermittency
The average capacity factor for the EU is only around 2,000 hours per year for wind energy and 1,200 hours for photovoltaics, i.e. 80% of the time they do not produce the power for which they were designed. Analysing the burden factors in France, Spain and Italy, over a year, we observed that intermittency is a serious handicap for the development of intermittent renewable energy in a free market.
Table 1 – Electricity production load factor from wind and solar PV in France (TEN data), Spain (REE data) and Italy (Terna data) in 2019
Currently, this intermittency is offset by the commissioning of gas-fired power plants, which greatly increases the carbon footprint of the whole process. The only remedy is to try to store large amounts of intermittent energy when it is overabundant, and to release it as needed.
Several options are possible: re-pumping to dams, compressed air, batteries and hydrogen production (by electrolysis) are most often cited. The first three options having shown their limits of capacity, technical and economic feasibility and acceptability, remains the hydrogen option … unless we continue to use natural gas as backup plants, as is currently being done.
Chemistry teaches why commercial hydrogen is not produced by water electrolysis
It should be noted that the idea of producing hydrogen from wind turbines is not new, since the British scientist John Haldane, who feared the end of coal in his country, had already proposed it in 1923. If that did not happen, it is due to good reasons.
The hydrogen molecule is not used as an energy vector because it has been ascertained as being more useful as a basic molecule in chemistry for more than a century. The annual production of hydrogen is about 115 million tons, much of which is used by the chemical industry to produce ammonia, which in turn will be used to make fertilisers for agriculture, feeding the world’s population. Hydrogen also serves in petrochemicals to transform low-hydrogenated hydrocarbons into lighter petroleum products for the multiple uses of petrochemicals. An important fraction of it is also used to remove sulphur from petroleum products, thus avoiding air pollution this way.
Given that it was in 1766 that the chemist Henry Cavendish discovered hydrogen, and its importance to chemistry, it will not be surprising to learn that the means of producing this molecule have been the subject of numerous studies and comparisons. Since then, know-how has continued to improve and for decades, global hydrogen production has rested, and still relies, on the transformation of hydrocarbons by reaction with water vapour, the process being called reforming. Since natural gas is the most widely used feedstock, the commonly used technical term is Steam Methane Reforming (SMR).
But why did this reaction take hold and not that of water electrolysis? Even in the 1970s and 1980s, when there was enthusiasm for cheap electric power produced by nuclear powerplants, it was not envisaged. Yet we have all seen our chemistry teacher demonstrating water electrolysis on his mat; so, it is apparently something simple, since you can do it in a school laboratory! That has not been done – and will not happen – because the electrolysis is too energy-intensive.
Energies of formation
Let’s see some enthalpic calculations related to hydrogen manufacturing
Reaction 1 (electrolysis):
H20 (l) → H2 + ½ O2 ΔR1 = 285.8 kJ [1]
to produce one hydrogen mole, i.e. (285.8 × 1,000) / (2 × 3,600) = 39.7 kWh/kg of hydrogen)
Reaction 2: (assuming water vapour is an otherwise lost vapour from the petrochemical complex)
CH4 + 2 H20 (v) → CO2 + 4H2 ΔR2 = 164.7 kJ [2]
to produce four hydrogen moles, or 41.2 kJ per mole
Reaction 2 bis: (same as 2, but starting from liquid water)
CH4 + 2 H20 (l) → CO2 + 4H2 ΔR2bis = 252.7 kJ [2 bis]
to produce four hydrogen moles, or 63.2 kJ per mole.
As a result, by hydrogen mole, we get the following ratios:
Ratio (reaction 1)/(reaction 2): 285.8/41.2 = 6.94 = ~ 7
Ratio (reaction 1)/(reaction 2 bis): 285.8/63.2 = 4.52 = ~ 4.5
We can see that producing hydrogen from liquid water requires 4.5 times more energy per electrolysis than by SMR. If, as is often the case, in petrochemical plants there is steam generated by other chemical productions, the use of this fatal heat will cause the electrolysis to be 7 times more energy-intensive than the SMR. These are not small margins, but are insurmountable gaps. Hydrogen produced by electrolysis of water therefore has no thermodynamic justification, whether the electricity is of renewable origin (to produce hydrogen described as green) or not.
Using the SMR reaction for energy purposes?
But then if we want a hydrogen-based energy, why not produce it from methane? This route is called ‘blue’ in the jargon of ‘hydrogenists’. Is there an advantage to turning methane into hydrogen and then burning it?
Here again, an enthalpic calculation of reaction heat will enlighten us.
Reaction 4: (direct combustion of methane)
CH4 + 2 O2 → CO2 + 2H20 (v) ΔHR4 = – 802.5 kJ [4]
Reaction 5: (steam reforming of methane followed by hydrogen combustion)
CH4 + 2 H20 (v) → CO2 + 4H2 ΔR2 = 164.7 kJ
Followed by hydrogen combustion
4H2 +2O2 → 4H20 (v) ΔR1 = – 967.2 kJ
Which gives in total:
CH4 +2O2 → CO2 + 2 H20 ΔR5 = – 802.5 kJ [5]
We can see that [5] <> [4] <> [2] + 4*[1]
This calculation shows that the (theoretical) energy generated by the two streams is perfectly identical. In fact, this is nothing else than an illustration of the principle of the initial and final state, well known in thermodynamics. Logically, therefore, there is no energy advantage in converting methane into hydrogen for combustion compared to its direct combustion. The same conclusion is reached in terms of the amounts of CO2 released, the equations [4] and [5] being perfectly identical. Worse, in addition to the increased investments and operating costs required for the hydrogen combustion chain, hydrogen being produced from methane, as compared to the direct combustion of methane, the efficiency of the steam reforming reaction is less than 100%, making the idea of the ‘blue hydrogen’ from methane chain even less attractive.
‘Green’ hydrogen manufactured by electrolysis
In principle, the operation is white, since it is the same reaction performed in the opposite direction (decomposition and then synthesis of water). But electrolysis is made from liquid water and combustion generates water vapour, so the maximum theoretical efficiency of the chain is LHV/HHV [c] (241.8/285.8 kJ) or ~ 84%. Currently, industrial yields are in the range of 50–70%[1]. The complete electrolysis chain followed by combustion is therefore in fact energy deficient, even if theoretically it should not consume energy. In other words, it is pure energy wastage.
At what cost?
There is a great deal of uncertainty about the costs of the various methods for producing hydrogen. This shows that some of the solutions proposed are still in the developmental stage. The following table, prepared from a detailed study made by the World Nuclear Association[2] (February 2021), indicates highly variable costs (estimated for 2030 or 2050), ranging from 0.7 to 1.6 euros/kg H2 for production by SMR, and in case of capture and storage of CO2released by the SMR reaction, the cost jumps to 1.3 to 2.5 euros/kg. For electrolysis from renewable electricity (green hydrogen), estimates range from single to more than double ($2.5 to $6.8/kg H2), with a prospect of reduction down to $1.4/kg in 2030, depending on the cost of electricity and the electrode catalysts used. It is interesting to remember here that the price of electricity varies enormously from country to country and is strongly correlated with the percentage of intermittent energy in the energy mix, as shown in Figure 1 below. In other words, we are trying to promote the intermittent energy sector, and limit the effect of its intermittency (which can cause economically and socially devastating blackouts), using an energy vector that is far too expensive at the moment, because it is energy-intensive, and whose cost will inevitably increase with greater market penetration by said intermittent energies.
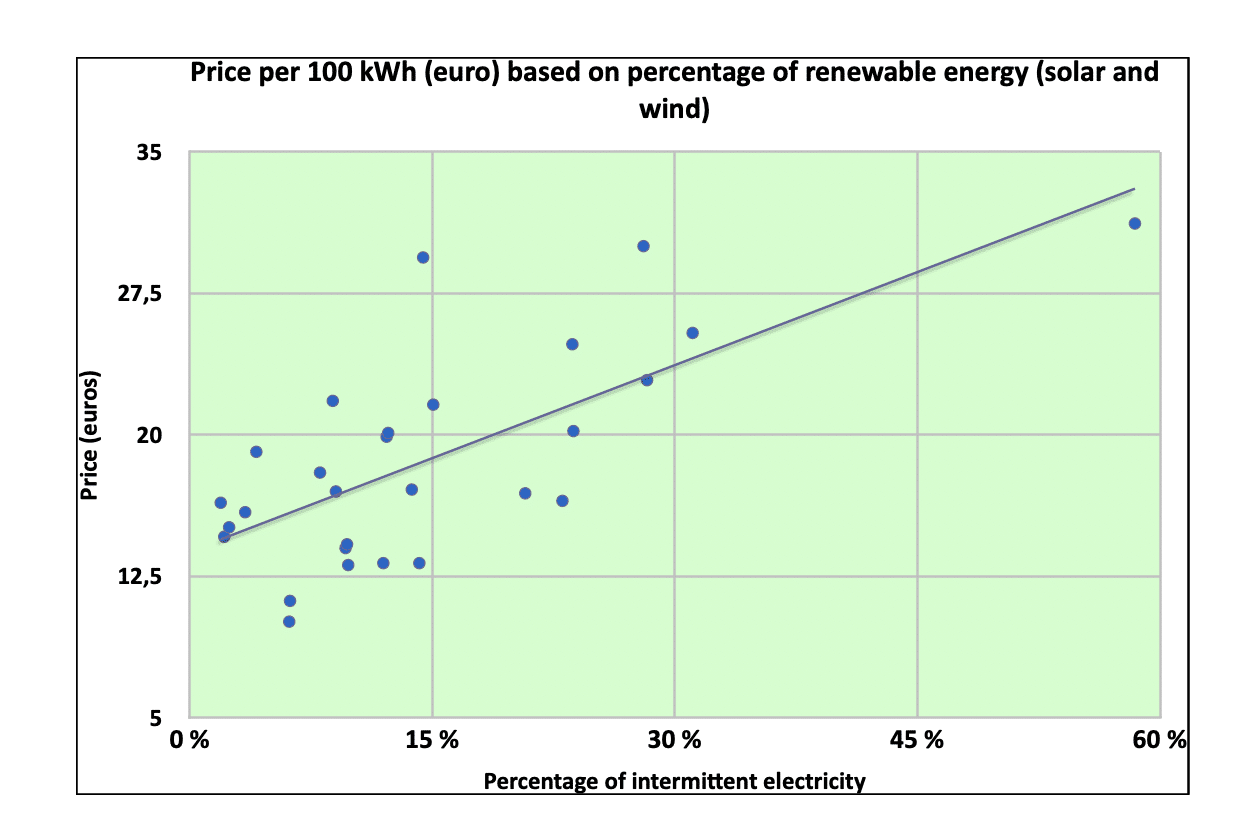
Table 2 – World Nuclear Association cost estimates of hydrogen production2
(*) HTSE = High Temperature Steam Electrolysis) [associated with a nuclear power plant]
For the rest of the demonstration, let’s take the following estimated values: 1 euro/kg for the SMR, 2 euros/kg for the SMR with CO2 capture and storage, and for the electrolysis between 2.4 and 7.5 euros/kg.
But what if we penalise carbon?
The article cited in reference to the beginning of this text is entitled ‘CO2 must become more expensive’. Let’s see, based on some basic calculations, what its cost should be, by penalising the CO2 emission produced by the SMR reaction, in order to bring the two methods, SMR and electrolysis, to break even.
It should be noted beforehand that it is always possible to capture and store the CO2 produced by the SMR reaction (blue hydrogen line), which, according to the previous table, generates an additional cost of around 1 euro/kg of hydrogen produced.
Based on stoichiometry, reaction 2 (or 2 bis) produces for 16 kg of methane, 8 kg of hydrogen, but also 44 kg of CO2, or 5.5 kg of CO2/kg of hydrogen.
Table 3 – Carbon Cost Required for reaching break-even between SMR and electrolysis
The price of CO2, which could offset the price difference between the two processes, would therefore fall within a price window ranging from 250 euros/t to 1,200 euros/t, a range that exclude this solution for the time being. Cost projections to 2050 bring this cost down to 1,200 euros/t, leading to a carbon cost for breakeven of 36 euros/t. But for that, the capacity factor of the renewable energy plants needs to be around 4,200 hours per year, a performance that could only be achieved with an ‘offshore’ wind turbine located in the best[3] wind-exposed areas, and by keeping the current price of electricity.
Figure 2 shows the very high volatility of the carbon market in Europe [4]. The average historical cost of CO2 on the carbon market has ranged progressively from 5 euros to 40 euros/t over time, with a significant increase at the end of 2020; the projected cost in the United States, under the Biden Administration, is expected to fall in the $47–50/t range (€40 – 42).
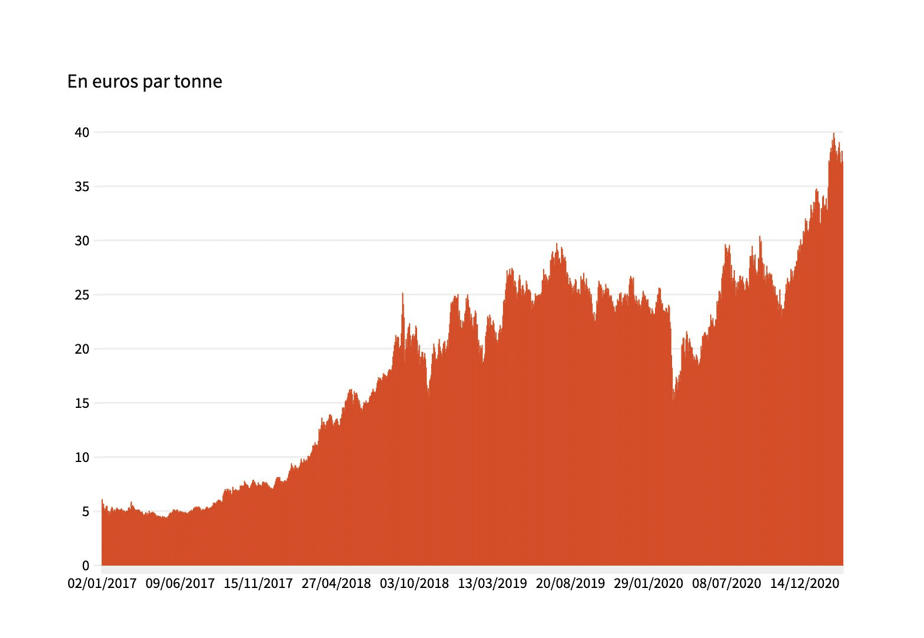
As a defensive tactic, it is likely that SMR hydrogen producers will opt for the capture and sequestration of CO2 over the next two decades, for purely economic reasons (their cost ranges then between 1,500 and 2,000 euros/t); which will nevertheless allow them to maintain a competitive price advantage over green hydrogen producers. Consequently, promoting hydrogen as an energy vector will not result in using ‘green’ hydrogen, but ‘blue’ hydrogen. Ironically, to decarbonise the EU economy, hydrogen will be produced from natural gas (methane), rather than by electrolysis. And this even though direct combustion of methane could be achieved at a lower cost, with much better energy efficiency, and with the same overall decarbonisation impact than burning “blue hydrogen. This is what happens when ideology supersedes logic (or knowledge?): while the EU keeps stating that we must impose energy efficiency, the exact opposite will happen.
These few basic notions of chemistry and physics teach us that water electrolysis has no chance of supplanting hydrogen production from methane, especially as the price of natural gas will fall in the coming years, as is already the case in the United States, given its abundance and the dynamics of energy geopolitics. Precisely, for this same reason, imposing by regulations exaggerated higher carbon prices in the EU, needed for making the electrolysis sector economically competitive with the SMR sector, while this will not be applied elsewhere, is suicidal. It can only be envisaged in a fortress Europe cut off from the evolution of industrial chemistry in the rest of the world; because the amount of hydrogen produced for chemical purposes will continue to grow, and in a free and open global market, there can only be one same price for a product. Hydrogen manufactured for energy is therefore nothing more than an illusion, has no future and will remain an energy of the future for the foreseeable future[5]. Burning hydrogen is as inept as burning Louis Vuitton handbags for heating.
Legislative constraint?
Green hydrogen is fascinating because it is a gas produced from water that burns by producing only water vapour. However, several technological, legislative, regulatory and economic challenges need to be addressed. For example, legislation will have to be enacted on defining the exact nature of electrolysers followed by fuel cells: are they consumers or generators of electricity? Also, if electrolysers are used to produce hydrogen further injected into a natural gas pipeline, it will impact the gas market, which is being developed in the EU, which will also require legislative adjustments. Interviewees from the Frankfurter Allgemeine Zeitung are quite right in saying that the future of hydrogen as energy will not depend on science: science has already demonstrated that it makes no sense from the point of view of macro-energy, that is, from the point of view of the overall energy balance. Only legislative constraints, together with the usual generous subsidies, can justify this energy aberration. The political world should be more careful before drawing plans on the comet: promising a miracle solution, at a cost impossible to achieve. And this, while respecting a totally unrealistic timetable for implementation, totally incompatible with the innumerable constraints of any order that must be overcome or circumvented, and of which apparently, politicians have not measured the magnitude, or worse, of which they seem to ignore the existence.
Moreover, before justifying such a hydrogen strategy on the pretext that we must decarbonise our economy, we should also consider that the EU in 2030 will only contribute 6% to global CO2 emissions; even a zero European emission in 2050 will have a marginal impact on the global balance sheet. Given the hundreds of millions of people who do not have access to energy, is a self-referenced European policy, suggested by lobbyists, who take advantage of this policy based solely on ideological considerations, sufficient and adequate, or should other innovative scientific approaches be considered rather than purely legislative solutions?
[a] Professor of energy geopolitics at ULB and President of the European Society of Engineers and Industrialists
[b] Professor, Director of the Centre for Artificial Intelligence and Data Processing (Swiss School of Management)
[c] LHV = Lower Heating Value ; HHV = Higher Heating Value
[1]https://www.scribd.com/document/407794017/Comparasion-of-Different-H2-Companies
[2] www.world-nuclear.org/information-library/energy-and-the-environment/hydrogen-production-and-uses.aspx
[3] IRENA (2019), Hydrogen: A renewable energy perspective, Abu Dhabi, https://www.irena.org/-/media/Files/IRENA/Agency/Publication/2019/Sep/IRENA_Hydrogen_2019.pdf
[5] Samuel Furfari, “Hydrogen Utopia,” KDP, 2020, ISBN 9798676846862
Follow up with Samuel Furfari, a good read on the same topic
This post is also available in: FR (FR)
Catalyst inducing conversion is a consistent theme of chemistry/technology. Hydrolysis of water is an energy panacea!
Abandoning electrolysis of water prematurely might be foolish. Economics change!
Given electron overloads in the grid with wind and alternative energies, this might be the time to experiment!